Download PDF
Aeroelastic Investigation of Wind Turbine Blades Using Computational Fluid Dynamics
Technology Category
- Drones - Multirotor Drones
- Sensors - Level Sensors
Applicable Industries
- Electrical Grids
- Renewable Energy
Applicable Functions
- Maintenance
- Product Research & Development
Use Cases
- Digital Twin
- Virtual Reality
The Challenge
The increasing trend in wind power technology is to enhance power output through an increase in rotor diameter. However, as the rotor diameter increases, aeroelastic effects become increasingly important in the design of an efficient blade. A detailed understanding of the fluid elastic coupling can lead to improved designs, yielding more power, reduced maintenance, and ultimately leading to an overall reduction in the cost of electricity. Current wind turbine design practices use desktop engineering tools such as FAST and ADAMS to provide information about the aero-elastic behavior of the turbines. However, each of these techniques has its own advantages and disadvantages. An evolving approach for generating performance data on wind turbine rotors is through the use of Computational Fluid Dynamics (CFD).
About The Customer
The customer in this case study is not explicitly mentioned. However, it can be inferred that the customer would be any organization or entity involved in the design, production, and operation of wind turbines. This could include wind power technology companies, renewable energy providers, and potentially government bodies or research institutions involved in the development and regulation of wind power technology. The customer would be interested in this study as it provides a detailed methodology for improving the design and efficiency of wind turbine blades, potentially leading to increased power output, reduced maintenance costs, and an overall reduction in the cost of electricity generated by wind power.
The Solution
In this study, a high fidelity Computational Fluid Dynamics (CFD) methodology is presented for performing fully coupled Fluid-Structure Interaction (FSI) simulations of wind turbine blades and rotors using a commercially available flow solver, AcuSolve. The fully coupled fluid/structure interaction problem is simulated using a modal superposition approach. This technique, known as Practical Fluid Structure Interaction (or P-FSI) requires the eigenvalues and eigenvectors of the structure as input to the CFD model. Once this information is provided, AcuSolve is able to independently compute the structural deformation in response to the fluid forces on the wetted surfaces. The starting point for this analysis is a CAD model of the 13.2 MW blade design. The geometric model is created based on the specified airfoil sections that make up the blade geometry. For the CFD side of the analysis, the bounding fluid volume around the rotor is created using a simple cylindrical solid region.
Operational Impact
Quantitative Benefit
Related Case Studies.
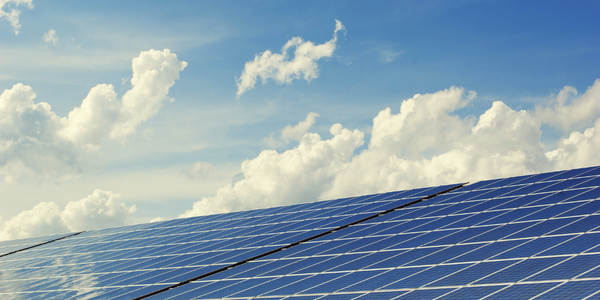
Case Study
Remote Monitoring & Predictive Maintenance App for a Solar Energy System
The maintenance & tracking of various modules was an overhead for the customer due to the huge labor costs involved. Being an advanced solar solutions provider, they wanted to ensure early detection of issues and provide the best-in-class customer experience. Hence they wanted to automate the whole process.
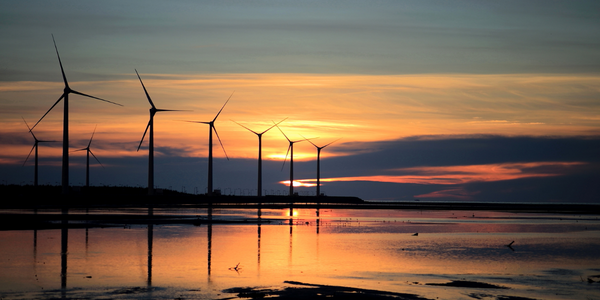
Case Study
Vestas: Turning Climate into Capital with Big Data
Making wind a reliable source of energy depends greatly on the placement of the wind turbines used to produce electricity. Turbulence is a significant factor as it strains turbine components, making them more likely to fail. Vestas wanted to pinpoint the optimal location for wind turbines to maximize power generation and reduce energy costs.
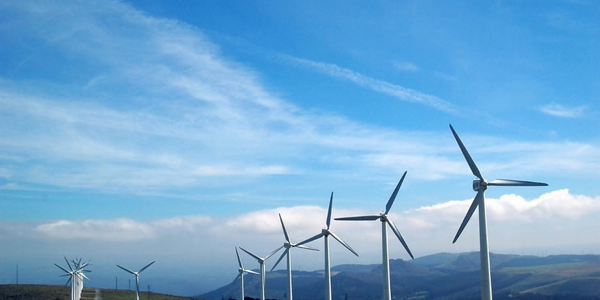
Case Study
Siemens Wind Power
Wind provides clean, renewable energy. The core concept is simple: wind turbines spin blades to generate power. However, today's systems are anything but simple. Modern wind turbines have blades that sweep a 120 meter circle, cost more than 1 million dollars and generate multiple megawatts of power. Each turbine may include up to 1,000 sensors and actuators – integrating strain gages, bearing monitors and power conditioning technology. The turbine can control blade speed and power generation by altering the blade pitch and power extraction. Controlling the turbine is a sophisticated job requiring many cooperating processors closing high-speed loops and implementing intelligent monitoring and optimization algorithms. But the real challenge is integrating these turbines so that they work together. A wind farm may include hundreds of turbines. They are often installed in difficult-to-access locations at sea. The farm must implement a fundamentally and truly distributed control system. Like all power systems, the goal of the farm is to match generation to load. A farm with hundreds of turbines must optimize that load by balancing the loading and generation across a wide geography. Wind, of course, is dynamic. Almost every picture of a wind farm shows a calm sea and a setting sun. But things get challenging when a storm goes through the wind farm. In a storm, the control system must decide how to take energy out of gusts to generate constant power. It must intelligently balance load across many turbines. And a critical consideration is the loading and potential damage to a half-billion-dollar installed asset. This is no environment for a slow or undependable control system. Reliability and performance are crucial.
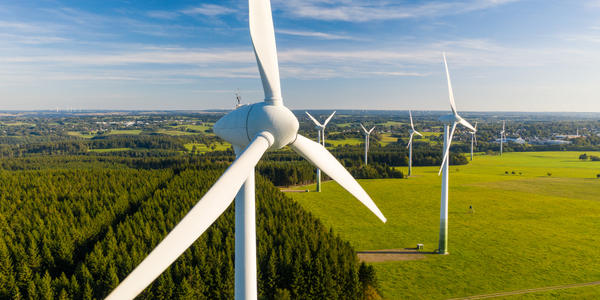
Case Study
Remote Monitoring and Control for a Windmill Generator
As concerns over global warming continue to grow, green technologies are becoming increasingly popular. Wind turbine companies provide an excellent alternative to burning fossil fuels by harnessing kinetic energy from the wind and converting it into electricity. A typical wind farm may include over 80 wind turbines so efficient and reliable networks to manage and control these installations are imperative. Each wind turbine includes a generator and a variety of serial components such as a water cooler, high voltage transformer, ultrasonic wind sensors, yaw gear, blade bearing, pitch cylinder, and hub controller. All of these components are controlled by a PLC and communicate with the ground host. Due to the total integration of these devices into an Ethernet network, one of our customers in the wind turbine industry needed a serial-to-Ethernet solution that can operate reliably for years without interruption.
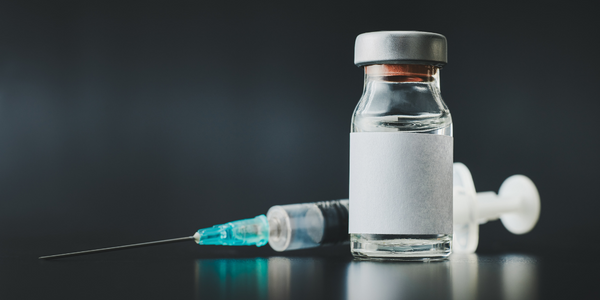
Case Study
Temperature monitoring for vaccine fridges
Dulas wanted a way to improve the reliability of the cold chain, facilitating maintenance and ensuring fewer vaccines are spoiled. Dulas wanted an M2M solution which would enable them to record and report the temperature inside vaccine refrigerators.
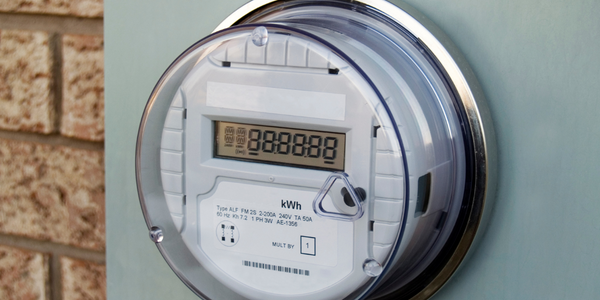
Case Study
Hydro One Leads the Way In Smart Meter Development
In 2010, Ontario’s energy board mandated that time-of-use (TOU) pricing for consumers be available for all consumers on a regulated price plan. To meet this requirement, Hydro One needed to quickly deploy a smart meter and intelligent communications network solution to meet the provincial government’s requirement at a low cost. The network needed to cover Hydro One’s expansive service territory, which has a land mass twice the size of Texas, and its customers live in a mix of urban, rural, and remote areas, some places only accessible by air, rail, boat or snowmobile. Most importantly, the network needed to enable future enterprise-wide business efficiencies, modernization of distribution infrastructure and enhanced customer service. To meet these needs, Hydro One conceptualized an end-to-end solution leveraging open standards and Internet Protocols (IP) at all communication levels. The utility drew upon industry leaders like Trilliant to realize this vision.